Sound, light sources and the thrill of glimpsing the future
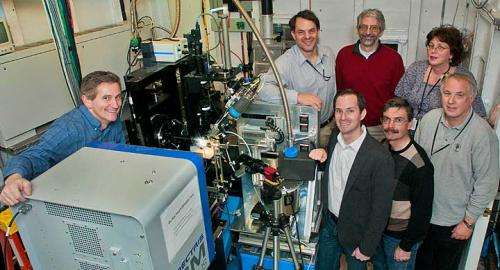
Scientific research is a process fraught with fits and starts, dead-ends, dashed dreams, unexpected turns, and the occasional exhilarating insight. As scientists, many of us continue along our career path in part because of those rare moments of joy felt when contributing to the frontiers of science. We experienced one of those rare moments this past January at the National Synchrotron Light Source (NSLS) X25 beamline, when we successfully tested a new method to collect data from protein crystals—by using sound waves to eject the crystals through the air into an X-ray beam.
For decades now, preparing microscopic protein crystals to collect data was done typically by hand with a microscope, and lassoing a crystal out of its liquid environment required great skill. After that was done, the sample would be transported from the lab to a beamline end station and then mechanically transferred into the X-ray beam, where we collected data to determine the atomic structure of complex macromolecules.
In contrast, this new method—called acoustic droplet ejection (ADE)—employs pulsed sound waves to eject crystals encased in a droplet from their source into the X-ray beam. This can be done with great precision, at high speeds, and without touching the crystals at all. back in 2008, but we were overcome with joy when, last month, we observed the first X-ray diffraction pattern from crystals with our new, automated ADE method.
ADE in action at Brookhaven Lab, and beyond
We tested our ADE system at the X25 beamline by depositing slurries of protein crystals—whose diameters are measured in millionths of a meter—onto a sheet of X-ray transparent plastic. With these protein crystals affixed to the plastic, we were able to confirm that the quality of data from the X-ray diffraction process was not compromised at all compared to traditional approaches.
Our ADE system enables us to mount a sample and record X-ray diffraction patterns every few seconds. This is significantly faster than the current methods that require several minutes per sample. When necessary, this ADE method is powerful enough to deliver hundreds of crystal-containing droplets every second.
Commercial ADE systems are available for drug discovery and genomics pipelines, and are large, boxy objects about half the size of a refrigerator. The system we constructed is much smaller and modular, making it the first ADE instrument that can work within the tight space constraints of most beamlines at a synchrotron. We anticipate that these methods will be vital for scientists at modern facilities such as the future National Synchrotron Light Source II (NSLS-II) and the existing Linac Coherent Light Source (LCLS) at SLAC National Accelerator Laboratory.
Vaporization? the X-ray exposure challenge at modern light sources
At a proposed beamline at NSLS-II, an X-ray beam focused to one micrometer will destroy protein crystals in about 50 milliseconds, requiring very fast sample delivery and data collection. The problem intensifies at the LCLS, where individual sub-micrometer-sized crystals are imaged with X-rays pulsed at 70 quadrillionths of a second before the crystals are vaporized. The bottom line is that a lot of precious X-rays are lost without more efficient ways to deliver samples.
It's too early to test the ADE system at NSLS-II, but at the LCLS, our ADE-based injector will deliver microcrystals more than one hundred times per second, in synchrony with the facility's X-ray pulse structure. Our ultimate goal is to minimize the amount of sample needed by using drop-on-demand ADE methods, making sure that each droplet is hit by an X-ray pulse.
In addition to our collaborators at Brookhaven, we have expanded our team to continue experiments beyond the X25 beamline at NSLS. The larger group now includes staff members at the LCLS, Stanford Synchrotron Radiation Light Source, Lawrence Berkeley National Laboratory, and Labcyte Inc. This expanded group will provide critical expertise when we deploy our acoustic sample delivery methods at the LCLS in June 2013.
Provided by Brookhaven National Laboratory