A revolution in light at the small scale
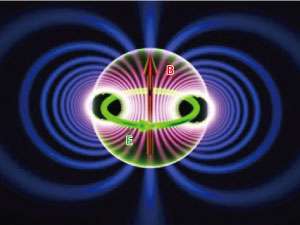
Light behaves in rather tame and predictable ways when interacting with everyday objects—it travels in straight lines, rebounds when it hits shiny surfaces, and gets bent by lenses. But weird and wonderful things start to happen when light interacts with very small objects. Nanoparticles, for example, which are collections of atoms as small as a virus, can act as mini-antennas, and small disks of silicon can set off strange 'modes' of light that render the disks invisible.
A new area of optics has emerged in recent years to study these strange phenomena. "Nanophotonics, a branch of optics dealing with light at nanoscale dimensions, has become a hot research topic over the last decade or so," notes Arseniy Kuznetsov of the A*STAR Data Storage Institute. "It holds a lot of promise for various new applications, ranging from high-speed information transmission and holographic display technologies to bioimaging and genome sequencing." Kuznetsov's team is leading developments in a subfield of nanophotonics, which could ensure its widespread practical application.
Light on tiny scales
Traditionally, nanophotonics has focused on tiny metal structures such as gold and silver nanoparticles. The oscillating electric field of light causes the free electrons in metals to oscillate collectively. At certain particle sizes, this can give rise to an effect known as surface plasmon resonance. Resonance is a general phenomenon in which a system exhibits a much larger response at certain frequencies—for example, an opera singer can cause a wine glass to shatter by singing at the pitch that it resonates at. Surface plasmon resonance refers to the specific resonance effect produced by surface plasmons, which are a collection of charged oscillations—the study of which is known as nanoplasmonics. While a very new research area, nanoplasmonic effects have been exploited for centuries—stained-glass windows in medieval cathedrals owe their color to surface plasmons excited in metal nanoparticles embedded in the glass.
Despite the high expectations for nanoplasmonics in areas such as information technology, security, energy, high-density data storage and the life sciences, it has resulted in relatively few practical applications. One reason for this disappointing outcome is that metal nanostructures lose a lot of light to absorption. "A deeper understanding of these resonances has brought a general understanding of major drawbacks related to unavoidable high losses in resonant metallic nanostructures," comments Kuznetsov. Furthermore, metals commonly used for plasmonics such as silver and gold are incompatible with standard methods for manufacturing semiconductor components, making them difficult to produce.
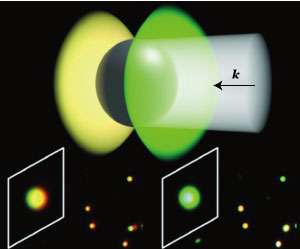
A quiet revolution
But now a quiet revolution is underway in this area. The focus is shifting away from metals and toward electrically insulating and partially insulating materials known as dielectrics and semiconductors, which are 'optically dense' so that light travels considerably slower in them than in air. Examples of such materials include the semiconductors silicon, germanium and gallium arsenide, and titanium dioxide.
"The shift from metals to dielectrics is already happening," says Kuznetsov. "Many leading teams in plasmonics have already started to work with resonant dielectric nanostructures."
Though still in its infancy, the transition has revealed many benefits. "After the demonstrations of resonances in dielectric nanoparticles in 2012, the field took off," says Kuznetsov. "Many advantages over conventional plasmonics have now been found."
Leading the way
Kuznetsov and his team at A*STAR are at the vanguard of this revolution. They employ a three-pronged approach. "In many cases, we generate a theoretical concept, show it in simulations and then demonstrate it experimentally. However, sometimes the reverse process occurs—unexpected experimental observations lead to theory development to provide their physical understanding," explains Kuznetsov.
The team members have realized some remarkable firsts in this young field. Âé¶¹ÒùÔºicist Boris Luk'yanchuk started the ball rolling in 2010 when he and colleagues in Germany published a seminal paper showing that, theoretically, silicon nanoparticles with sizes ranging from 100 to 200 nanometers might have both strong electric and magnetic resonances at visible-light frequencies—a low-loss alternative to plasmonic nanostructures. In a subsequent paper, Luk'yanchuk, together with researchers in Australia, proposed novel metal-dielectric hybrid structures where light could propagate due to interactions of magnetic moments, which is not possible in chains of metallic particles. Finally in 2015, the A*STAR group showed that similar types of optically induced interactions of magnetic moments exist in chains of silicon particles. "Such magnetic interactions of silicon particles can far outperform waveguides based on plasmonics and conventional silicon photonics," says Luk'yanchuk.
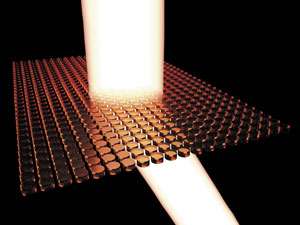
Luk'yanchuk, Kuznetsov and their team have experimentally demonstrated these resonances in silicon nanoparticles. The team was also the first to experimentally show unique directional light scattering by silicon nanoparticles, which demonstrates their promising nanoantenna properties. And the researchers were the first to experimentally show large enhancement of the electric and magnetic fields of light in close proximity to dielectric antennas made from two silicon nanoparticles placed very close to each other6.
According to Google Scholar, the papers describing these findings have been cited more than 1,000 times, reflecting the enormous impact that the team's work has had in the field. Such is their reputation in this area that a recent review they wrote on the emerging field was published in the prestigious journal Science.
In a 2015 study, the team, together with researchers from Australia and Germany, experimentally demonstrated a very unusual optical effect in nanoscale disks of silicon—patterns of radiation that do not emit or scatter light8. Such radiation modes could be used to produce tiny nanoscale lasers. The team has also showed how arrays of such silicon disks can precisely control the phase and amplitude of light, forcing it to bend, focus, or create high-resolution holographic images.
In 2016, the Institute of Âé¶¹ÒùÔºics Singapore awarded Luk'yanchuk the World Scientific Âé¶¹ÒùÔºics Research Award and Gold Medal for his outstanding contributions to physics research in the country. That same year, Kuznetsov was chosen as the recipient of the Institution of Engineering and Technology's A F Harvey Engineering Research Prize for "his outstanding contributions in the field of lasers and optoelectronics and his pioneering research on a new branch of nanophotonics: optically resonant dielectric nanostructures and dielectric nanoantennas."
A bright future
The team is excited about the potential of dielectric nanostructures. "We hope that resonant dielectric nanostructures will finally give rise to real-life applications from resonant nanophotonics," says Kuznetsov. They anticipate that many areas of technology could be strongly affected by this development.
"Three-dimensional holographic displays for smartphones and high-resolution virtual and augmented reality devices might be developed based on dielectric nanoantennas. Substrates containing resonant dielectric nanoparticles could make bioimaging and genome sequencing more efficient and faster. And rapid computers based on light may appear with resonant dielectric nanoparticle components inside," says Kuznetsov. "Some of these new and amazing applications may become reality in the next 5 to 8 years," he predicts. While light may be predictable on large scales, the future is looking anything but tame for this emerging technology.
More information:
Andrey E. Miroshnichenko et al. Optically Induced Interaction of Magnetic Moments in Hybrid Metamaterials, ACS Nano (2012).
Arseniy I. Kuznetsov et al. Magnetic light, Scientific Reports (2012).
Yuan Hsing Fu et al. Directional visible light scattering by silicon nanoparticles, Nature Communications (2013).
Reuben M. Bakker et al. Magnetic and Electric Hotspots with Silicon Nanodimers, Nano Letters (2015).
Arseniy I. Kuznetsov et al. Optically resonant dielectric nanostructures, Science (2016).
Andrey E. Miroshnichenko et al. Nonradiating anapole modes in dielectric nanoparticles, Nature Communications (2015).
Ye Feng Yu et al. High-transmission dielectric metasurface with 2Ï€ phase control at visible wavelengths, Laser & Photonics Reviews (2015).
Ramón Paniagua-DomÃnguez et al. Generalized Brewster effect in dielectric metasurfaces, Nature Communications (2016).
Journal information: Science , Scientific Reports , Nature Communications , ACS Nano , Nano Letters