May 9, 2019 feature
Generating multiphoton quantum states on silicon
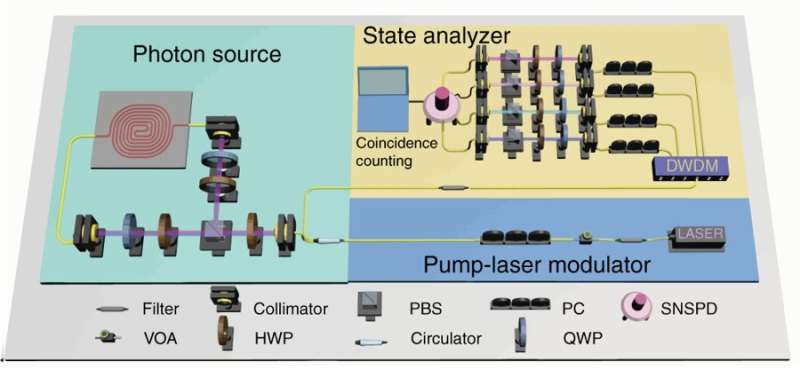
In a recent study now published in Light: Science & Applications, Ming Zhang, Lan-Tian Feng and an interdisciplinary team of researchers at the departments of quantum information, quantum physics and modern optical instrumentation in China, detailed a new technique to generate photon-pairs for use in quantum devices. In the study, they used a method known as to allow three electromagnetic fields to interact and produce a fourth field. The team created the quantum states in a silicon nanophotonic spiral to produce bright, tunable, stable and scalable multiphoton quantum states. The technology is comparable with the existing fiber and integrated circuit manufacturing processes to pave the way to engineer a range of new generation photonic quantum technologies for applications in quantum communication, computation and imaging. The multiphoton quantum sources detailed in the work will play a critical role to improve the existing understanding of .
The scientists generated multiphoton quantum states using a single-silicon nanophotonic waveguide and detected four-photon states with a low pump power of 600 µW to achieve experimental verified with . Zhang and Feng et al. recorded the quantum interference visibilities at a value greater than 95 percent with high fidelity. The multiphoton quantum source is fully compatible with on-chip processes of quantum manipulation and quantum detection to form large-scale (QPICs). The work has significant potential for multiphoton quantum research.
Multiphoton quantum sources are practical platforms for quantum , , and . Âé¶¹ÒùÔºicists have made great efforts to realize high quality, bright and scalable multiphoton quantum states in previous work, to activate powerful quantum technologies by multiplexing several biphoton sources to generate and . However, the efficacy of such multiplexing systems decreased with the number of entangled photons. At present, quantum photonic integrated circuits (QPCIs) and silicon-on-insulator (SOI) technology remain promising to realize high quality photon-pair sources.
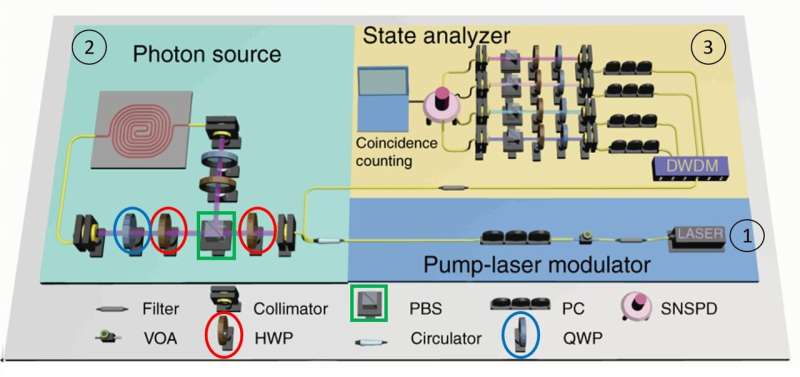
Silicon has several advantages as a substrate to implement QPICs, which include third-order of the material and ultra-high refraction index contrast for applications as SOI nanophotonic waveguides. Silicon is also compatible with complementary metal-oxide semiconductor (CMOS) processes—attractive for . While these advantages have allowed physicists to experimentally realize biphoton quantum sources, multiphoton quantum states on silicon still remain to be generated and reported.
In the present work, Zhang et al. generated four-photon polarization encoding quantum states using degenerated spontaneous four-wave mixing (SFWM) in a silicon spiral waveguide. The scientists first demonstrated biphoton Bell entanglement quantum states with high brightness (270 kHz) and a high (CAR, approximating 230) at a low pump power (120 µW). Thereafter, using the two biphoton Bell entangled states, Zhang et al generated the four-photon quantum state (with a pump power as low as 600 µW). The scientists projected this quantum product state to form a (GHz) state (i.e. a state in quantum information theory with at least three subsystems or particles) with 50 percent probability for further use in quantum information applications.
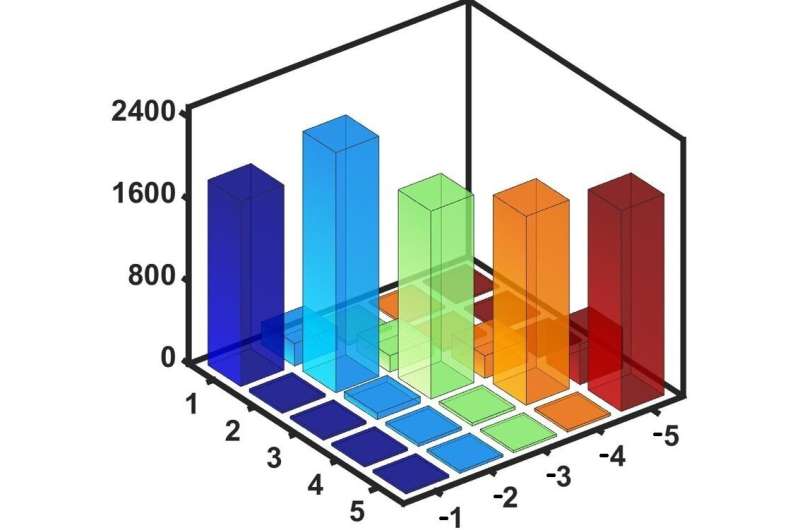
Zhang et al. constructed the experimental setup in three parts to contain the (1) pump-laser modulator, (2) the photon source and (3) the state analyzer. In the pump-laser modulator they introduced a linearly polarized pulse fiber laser as the pump source with a repetition frequency of 100 MHz and a pulse duration time of 90 femtoseconds (fs). The scientists coordinated the pump light to pass through a 100 GHz bandwidth pre-filter, followed by a polarization controller (PC) and an optical circulator to finally couple into the photon source. They calculated the coherence time of the pulse laser light to be 20 picoseconds (ps) after going through the 100 GHz bandwidth prefilter and the propagation loss in the silicon spiral waveguide was approximately 1 dB/cm.
Compared to multiphoton quantum states proposed with previous , the present work used a silicon nanowire source with near-zero broadband dispersion. The experimental setup with the did not demonstrate Raman scattering noise, which therefore greatly enhanced the number of photon pairs generated. Unlike with , Zhang et al. did not need to tune the operation wavelength in the experimental setup since they used silicon spiral waveguides instead. The scientists used grating couplers to couple-in the pump light and couple-out the generated photon pairs in the setup. As part of the photon source, Zhang et al. used a configuration with a Sagnac interferometer – a popular and self-stabilized scheme to states.
The experimental Sagnac interferometer contained two half-wave plates (HWPs), two quarter wave plates (QWPs), a polarization beam splitter (PBS) and the silicon spiral waveguide approximating a length of 1 cm to form a simple structure and compact footprint (170 x 170 µm2). The scientists used the combined HWP and QWP inserted between the PBS and the chip to control optical polarization and maximize the coupling efficiency of a photon pair. In the experiment, the on-chip generated photon pairs (idler and signal photons) could be superposed together in both directions (clockwise and counterclockwise) for output from the Sagnac loop. At this point, the scientists used a (fiber-optic transmission technique) to separate the signal and idler photons, or demultiplex them. They were thus able to freely select the photon pairs of any combined frequency channel via frequency detuning. The scientists noted that after going through the DWDM filters, the polarization and quantum states of the photon-pairs were unchanged.
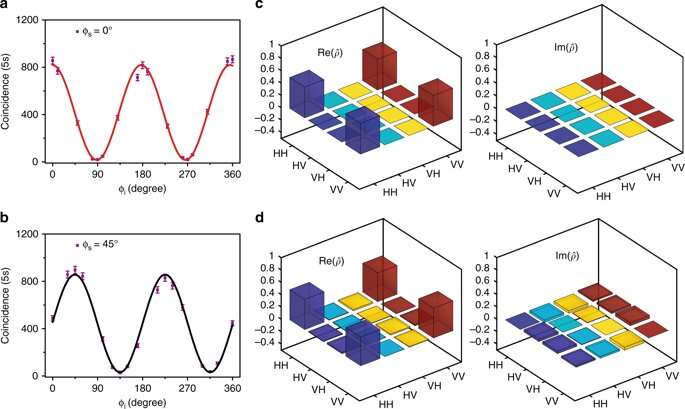
Zhang et al. then characterized the quality of the biphoton state generated in the experiment. For this, they selected five pairs of frequency channels used in the study to generate the signal and idler photons, to test the stability of the system. They measured the two-photon coincidences between different combinations of signal and idler channels and showed that the crosstalk was negligible for most frequency channels. After calculating the maximal polarization-entangled Bell state, they confirmed the existence of of the biphoton state. They credited the high coincidence-to-accidental ratio (CAR) observed to the ultralow nonlinear noise in the setup; necessary to generate multiphoton entanglement for further quantum information applications.
For complete characterization, the scientists connducted to reconstruct the experimental state density matrix architecture by completing multiple measurements of the relevant quantum state. The results confirmed that the generated biphoton quantum states are of high quality to approach the ideal maximally entangled states.
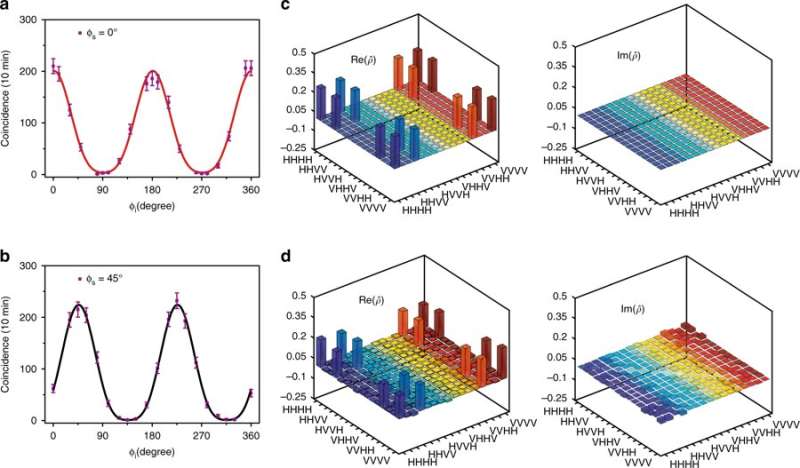
The scientists then conveniently generated multiphoton entangled states by multiplexing the biphoton states in different frequency channels. They obtained a fourfold coincidence rate and showed the observed four-photon state as the of two biphoton entangled Bell states. The four-photon interference patterns agreed with the theoretical prediction, to unfold differently from the previously observed biphoton entangled states. Based on the outcomes of a clear interference pattern and high interference visibilities, Zhang et al. verified the feasibility of the experimental technique to establish on-chip multiphoton quantum states. As before, the scientists obtained quantum state tomography of the four-photon quantum states to reconstruct the density matrix, yielding satisfactory results for further quantum information applications.
In this way, the scientists experimentally demonstrated the generation of four-photon quantum states using a silicon nanophotonic spiral waveguide. Zhang et al. aim to improve the photon collection efficiency to increase the number of entangled photons in the system in the future. The multiphoton quantum state source developed in the study is compatible with contemporary fiber and chip-scale architectures for large scale production. Zhang et al. therefore propose the integration of the attractive features as a scalable and practical platform for future quantum processing applications.
More information: Ming Zhang et al. Generation of multiphoton quantum states on silicon, Light: Science & Applications (2019).
Lucia Caspani et al. Integrated sources of photon quantum states based on nonlinear optics, Light: Science & Applications (2017).
Alán Aspuru-Guzik et al. Photonic quantum simulators, Nature Âé¶¹ÒùÔºics (2012).
Yin-Hai Li et al. On-Chip Multiplexed Multiple Entanglement Sources in a Single Silicon Nanowire, Âé¶¹ÒùÔºical Review Applied (2017).
Journal information: Light: Science & Applications , Nature Âé¶¹ÒùÔºics
© 2019 Science X Network