April 29, 2020 feature
Molecular engineering metal coordination interactions for strong, tough, fast-recovery hydrogels
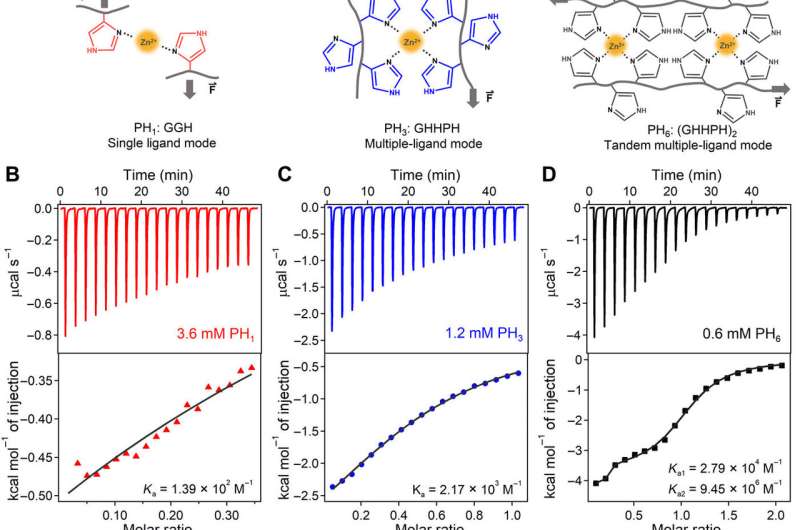
Load bearing tissues such as muscles and cartilages typically show high elasticity, toughness and fast recovery rates. However, combining such mechanical properties in the lab to build synthetic biomaterials is fundamentally challenging. In a new study now published on Science Advances, Wenxu Sun and a research team in physics, engineering mechanics and smart devices in China, developed a strong, tough and fast-recovery hydrogel. The team engineered the material using crosslinkers with cooperative dynamic interactions. They designed a -rich (10 amino acid chain) containing two tandem (consecutive) zinc (Zn) binding motifs to facilitate thermodynamic stability, stronger binding strength and faster binding rate of the construct, compared to single binding protein motifs or isolated ligand proteins. The engineered hybrid network hydrogels with the peptide zinc complex exhibited high stability, toughness and fast recovery in seconds. The research team expect the scaffolds to effectively manage load-bearing tissue engineering applications and function as building blocks for soft robotics. The new results provide a general route to tune mechanical and dynamic properties of hydrogels at the molecular level.
When we walk, our muscles, cartilage and tendons are subject to substantial mechanical loads, but biological tissues can recover rapidly to function reliably for many mechanical cycles. Bioengineers have explored soft hydrogels with muscle-like mechanical properties as , , , and in . They have devoted many efforts to enhance the mechanical strength and toughness of hydrogels by introducing mechanisms. Quick recovery is also a unique trait for load-bearing soft tissue, aside from mechanical strength and toughness, but synthetic hydrogels still lack a mechanism for quick recovery. For instance, traditional or hybrid network (HN) hydrogels with short polymer chains as sacrificial networks cannot typically recover soon—often taking a minutes to days.
The strength of a hydrogel depends on the lifetime of its , where slow binding/unbinding kinetics lead to strong hydrogels, while fast exchange rates yield soft ones. To obtain high strength and toughness the crosslinkers must be slow, but to achieve fast recovery, the crosslinkers must be dynamic with high rates of association and dissociation. To overcome this contradiction, naturally occurring load-bearing materials have used of weak interactions. In this work, Sun et al. similarly engineered hybrid network (HN) hydrogels with a specifically designed peptide-metal complex as the physical crosslinker. The team formed efficient metal binding sites in a peptide sequence to engineer hydrogels with the requisite characteristics.
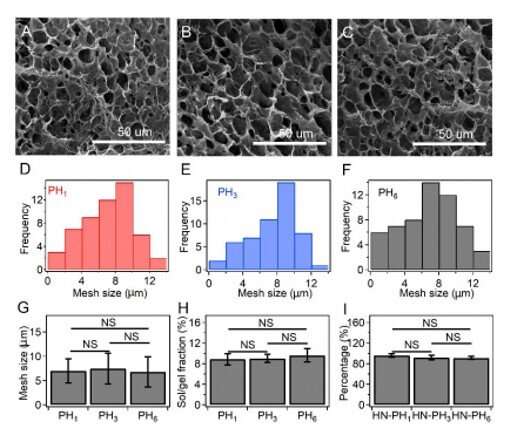
The team first designed three short histidine-rich peptides (HR-peptides) as ligands to bind with zinc ions (Zn2+) and construct HN hydrogels. They denoted the peptide sequences as PH1, PH3 and PH6 based on the number of linked histidines. Sun et al. synthesized the peptides using solid-phase peptide synthesis and purified it with high-performance . They observed the formation of Zn2+ histidine coordination complexes using ultraviolet (UV) and Raman spectroscopy. The specifically designed peptide sequence allowed synergistic and cooperative Zn2+ binding affinity, compared to peptides with random histidine residues on their sequences. The scientists studied the molecular mechanism of cooperative zinc ion binding to PH6 using , the results suggest conformal changes of the first coordination site of PH6 to be critical for cooperative binding and showed how structural changes favored additional Zn2+ binding.
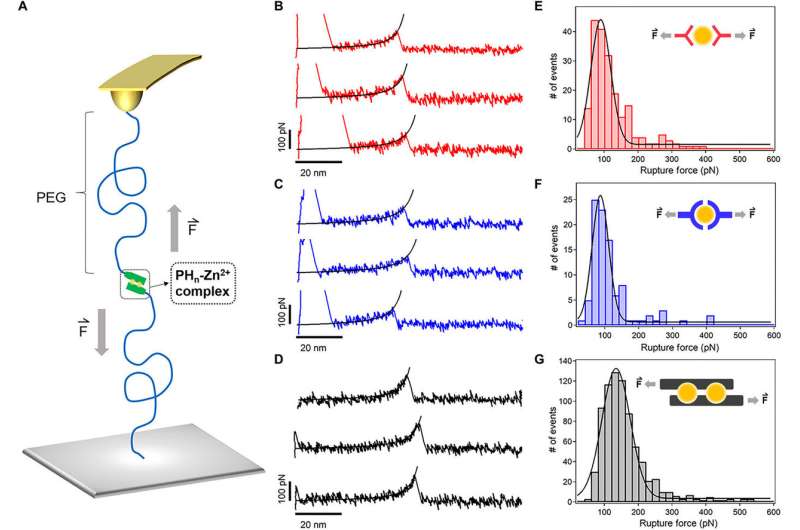
Sun et al. used advanced techniques such as atomic force microscopy (AFM)-based single- (SMFs) to measure the mechanical stability of the HR-peptide-Zn2+ complexes i.e. crosslinkers of the hydrogel at the molecular level. The average rupture forces were much higher for PH6 compared to other types of hydrogels, confirming the toughness of the hydrogel. The results showed that the mechanical stability of the metal-ligand complexes could be improved considerably based on the binding sites.
The team explored if changes to the intrinsic properties of crosslinkers could alter macroscopic mechanical properties of the hydrogel by preparing a series of hybrid network (HN) hydrogels. They used HR-peptide-Zn2+ as sacrificial crosslinkers and covalent bonds as permanent crosslinkers in the constructs and named the resulting hydrogels as HN-PH1, HN-PH3, and HN-PH6, based on the peptide sequence used. The network structures were similar in all three hydrogels but the HN-PH6 gel was more compressible compared to the others, while functioning effectively under stressful mechanical environments. Interestingly, the scientists could even twist the HN-PH6 hydrogel into a spiral shape and compress the material with a sharp blade without causing it permanent damage.
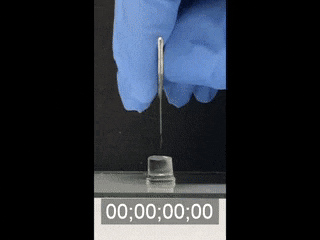
The team conducted on the gels and correlated the results on the bulk level with those at the molecular level, to show remarkably higher break strain, and toughness for the HN-PH6 gels. Sun et al. then examined the recovery property of the material based on loading-unloading cycles and found HN-PH6 gels to almost totally recover its macroscopic mechanical properties in minutes. However, if they cut up the HN-PH6 gels into pieces, the hydrogel could not self-heal since covalent crosslinkers do not reform after fracture. To understand the experimental outcomes, the research team also conducted theoretical analyses and proposed cooperative zinc binding on PH6 to be an important factor, among other factors to form strong and tough hydrogels with fast recovery rates.
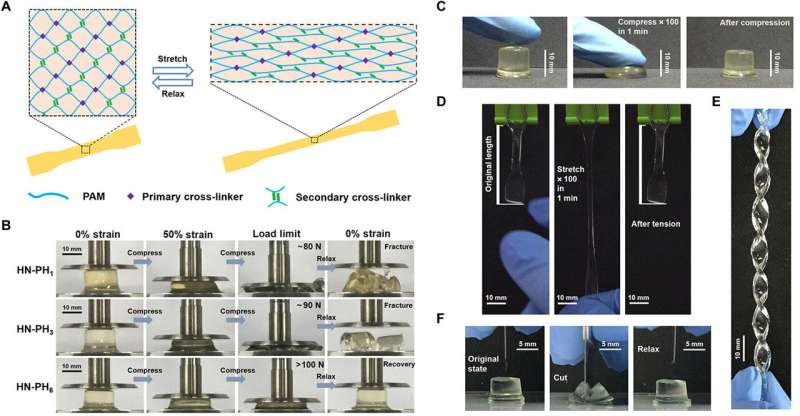
In this way, Wenxu Sun and colleagues developed a novel hydrogel material, bioinspired by histidine residues found in natural load-bearing materials. Combining such outstanding mechanical properties in the lab has remained a challenge due to the inability to effectively harness the unique metal ion binding properties that are encoded in natural proteins. In this work, Sun et al. used bioinspired Zn2+-binding peptide as crosslinkers to form the desired hydrogels at the molecular level, highlighting the importance of cooperative metal coordination during materials synthesis. They intend to examine additional mechanical features, such as , before conducting practical applications in tissue engineering.
More information: Wenxu Sun et al. Molecular engineering of metal coordination interactions for strong, tough, and fast-recovery hydrogels, Science Advances (2020).
C. Cvetkovic et al. Three-dimensionally printed biological machines powered by skeletal muscle, Proceedings of the National Academy of Sciences (2014).
Jeong-Yun Sun et al. Highly stretchable and tough hydrogels, Nature (2012).
Journal information: Science Advances , Proceedings of the National Academy of Sciences , Nature
© 2020 Science X Network