October 7, 2020 feature
Was the moon magnetized by impact plasmas?
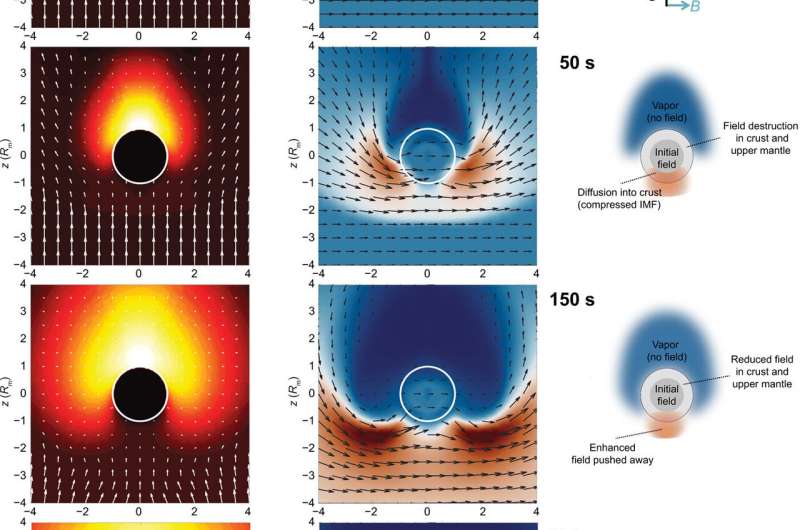
The moon, Mercury and many meteorite parent bodies contain a magnetized crust, which is commonly credited to an . A longstanding alternative hypothesis suggests the amplification of the interplanetary magnetic field and induced field of the crust (crustal field) via plasma generated through meteoroid impacts. In a new report now published on Science Advances, Rona Oran and a research team in the Departments of Earth and Planetary Sciences, Geosciences and Space Science in the U.S., Germany and Australia showed that although impact plasmas can transiently enhance the field inside the moon, the resulting fields were at least three orders of magnitude too weak to explain magnetic anomalies of the lunar crust. The team used magnetohydrodynamic and impact simulations alongside analytical relationships in this work to show the core dynamo (and not plasmas generated by asteroid impact) to be the only possible source of magnetization on the moon.
The lunar dynamo and lunar crust
The inductively generated magnetic fields in a fluid planetary interior is generated via the . The moon presently lacks a core dynamo magnetic field, but as of the , scientists have shown that the lunar crust contained . According to studies, the magnetizing field likely reached tens of microteslas more than 3.56 billion years ago, however, the origin of the strongest lunar crustal anomalies and their source of magnetization remain long-standing mysteries. Preceding studies of a fundamentally different non-convective dynamo mechanism on the moon.
More specifically, the hypervelocity resulting can vaporize and ionize lunar crustal materials to directly release plasma into the wind. Since the strongest and largest anomalies of the lunar crust are directly located at the antipodes (geographical sites) of , researchers hypothesize impact plasmas to have engulfed the moon and compressed the interplanetary magnetic field (IMF) to cause an enhanced crustal field at the antipode. Oran et al. addressed the existing gaps by introducing self-consistent modeling of post-impact plasmas and magnetic fields to explain field diffusion and dissipation inside the moon—alongside revised analytical considerations. To accomplish this, the team combined of basin excavation and vapor generation with (MHD) simulations.
The scientists used the to perform impact basin-forming simulations, a multimaterial, multirheology code in two dimensions (2-D). They also drove 3-D MHD (magnetohydrodynamic) simulations including the interaction of the moon, the solar wind and the vapor. During MHD simulations, Oran et al. used the (abbreviated BATS-R-US) code, capable of modeling the magnetic field evolution . They then focused on the of the moon—also known as the right eye of the fabled man in the moon; formed via an asteroid or protoplanet collision. The antipodal region of the Imbrium currently contains some of the observed from orbit. They simulated the impactor-based basin formation method, including vapor generation and basin excavation. The expanding impact plasma of the simulation created a magnetic cavity and enhanced the interplanetary magnetic field (IMF) at its periphery, causing the IMF carried by the wind to pile up against the vapor.
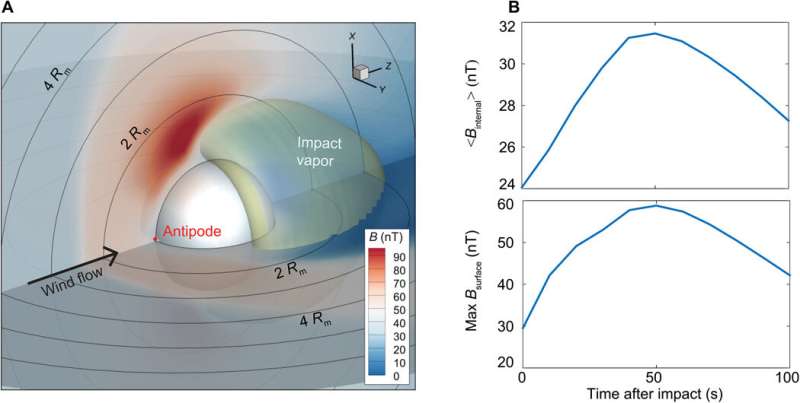
Studying the parameter space of different impact scenarios
At first, the moon's resistive outer layers destroyed the magnetic flux at a rate comparable to the rate of vapor expansion. This rate of loss of the magnetic field was consistent with theoretical estimations that contributed to remove magnetic energy from the system. The 3-D diffusion of the field in the mantel and crust allowed the field to slip around the core instead of being anchored within. The results did not indicate the conservation of magnetic energy or field convergence. The work further indicated that plasma amplified fields cannot account for crustal magnetization and the strongest amplification occurred far above the surface of the moon. An additional mechanism that could have limited the antipodal effect was magnetic reconnection, although the phenomenon did not occur due to the absence of antiparallel field geometry. Any magnetic flux pushed toward the antipode either dissipated inside the moon or was advected away by vapor.
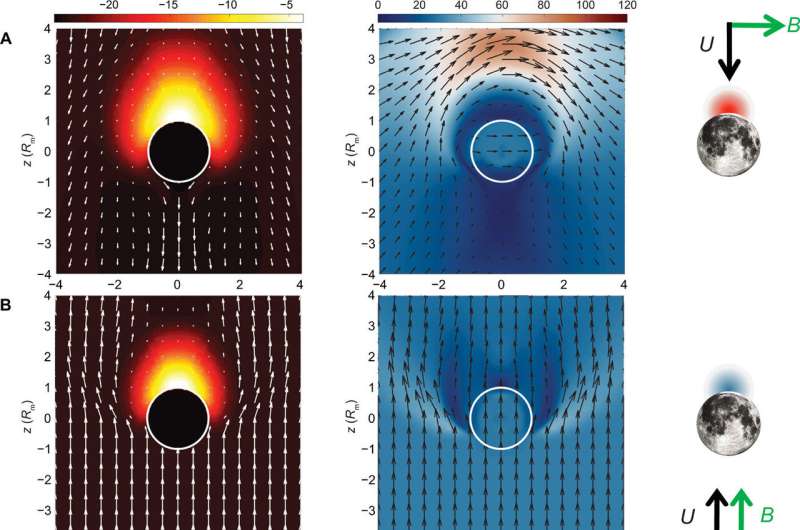
Oran et al. simulated seven additional choices for IMF (interplanetary magnetic field) detection including solar wind speed, impact location and impact cloud physical properties, with different combinations of parameters. They used several cases to explore alternative impact locations and relative orientations of the IMF and solar wind velocity. The largest overall amplification in the crest occurred in cases where the impact location and relative orientation of the IMF and solar wind velocity were similar.
Field enhancement due to vapor expansion into the solar wind
The MHD (magnetohydrodynamic) simulations showed how vapor expansion enhanced the interplanetary magnetic field (IMF) carried by the solar wind, presenting an obstacle to the wind, and causing de-acceleration and piling up. The source of the compressed IMF magnetic energy contained bulk kinetic energy of the upstream wind and the level of amplification was consistent with pile-up regions on comets and the ionosphere of Venus, while lower than the IMF compression ratio estimated for . The team also found the resistivity of the crust to be the main factor inhibiting magnetic field enhancement inside the moon. The magnetic field evolution occurred on a complex structure as reflected in the simulations, leading to the removal of flux from the crust and upper mantle, where the moon crust effectively reduced the magnetic energy on exposure to a magnetic cavity. This unexpected outcome was due to vapor expansion that occurred after impact, causing the incoming interplanetary magnetic field to change direction and gradually magnetically isolate the moon from the interplanetary magnetic field.
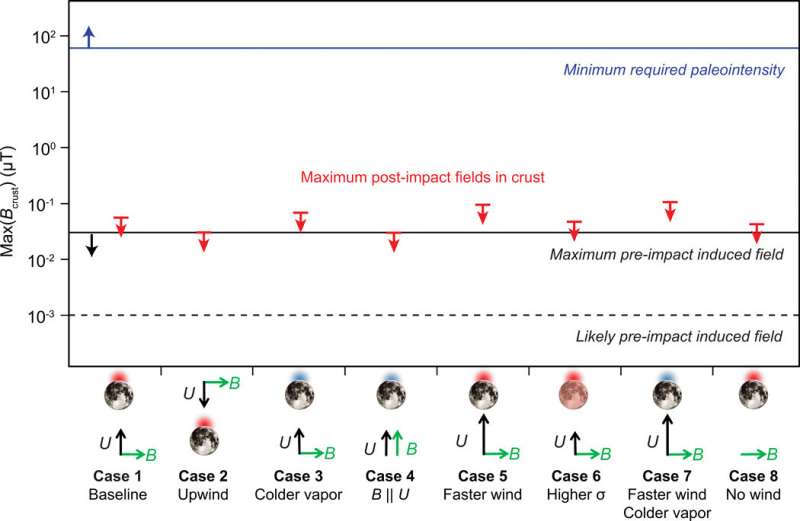
The impact-amplified magnetic field hypothesis is a leading alternative to a core dynamo origin of crustal magnetization in the moon and other interplanetary bodies. However, this work showed how such fields are too weak to explain the strong lunar crustal anomalies and of Apollo samples. Oran et al. therefore support the proposal of lunar paleomagnetism as a record of dynamo action on the moon. Impact plasmas may still be a viable mechanism to magnetize some regions of the crust if they are formed in the presence of a pre-existing core-dynamo field on the moon, such interactions remain to be further investigated with magnetohydrodynamic simulations.
More information: Rona Oran et al. Was the moon magnetized by impact plasmas?, Science Advances (2020).
Benjamin P. Weiss et al. The lunar dynamo, Science (2014).
C. A. Dwyer et al. A long-lived lunar dynamo driven by continuous mechanical stirring, Nature (2011).
Journal information: Science Advances , Science , Nature
© 2020 Science X Network