Understanding randomness: Researchers visualize decision-making in nanomagnetic structures
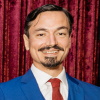
Robert Egan
associate editor

As the world's energy needs for computing and artificial intelligence continue to increase, developing alternative low-power solutions to traditional computing becomes crucial. Devices that reliably generate randomness, or stochasticity, are vital for many computing applications.
In new research, scientists at the U.S. Department of Energy's (DOE) Argonne National Laboratory have unveiled a novel approach to understanding stochasticity in tiny magnetic structures. Their work explores the intricate decision-making processes of nanomagnetic Galton boards, a modern take on a classical concept in statistics and computing.
Their insights, in Âé¶¹ÒùÔºical Review Letters, have the potential to transform computing architectures, leading to more sophisticated neural networks that can learn and adapt like the human brain, as well as enhancing encryption technologies to secure data against increasingly complex cyber threats.
A modern twist on a classic concept
A Galton board, named after British mathematician Sir Francis Galton, is a device that demonstrates statistical principles using a triangular array of pegs. As balls fall through the grid, they randomly bounce left or right, eventually landing somewhere along the bottom.
This process illustrates the central limit theorem by showing how, as balls fall through the board, most pile up toward the center, creating a bell-shaped curve that resembles a normal distribution. It shows how random processes can lead to predictable patterns.
In previous work, researchers from Université Paris-Saclay in France built on this classical concept and developed a nanomagnetic version of the Galton board. Instead of pegs, these boards use tiny magnetic structures made from a nickel-iron alloy. Instead of balls, they use domain walls, which are boundaries that separate regions with different magnetic orientations within a material.
These domain walls are injected into the device and guided through the structure by an applied magnetic field. As they approach a junction, they must make random decisions to move left or right, mimicking the classical Galton board process.
"The nanomagnetic Galton board is a fascinating adaptation of a classic concept, allowing us to explore randomness at the nanoscale," said Hanu Arava, a materials scientist at Argonne.
The path that each domain wall takes at each junction is random due to small variations in magnetic properties and other external influences. This randomness, a controlled form of stochasticity, can be harnessed for computation. Until now, the exact reasons behind the randomness in magnetic domains have not been fully understood.
Visualizing the invisible
Argonne researchers have visualized the decision-making process of domain walls in real time using Lorentz transmission electron microscopy. This state-of-the-art imaging technique allowed them to directly observe how domain walls navigate within the nanomagnetic structures.
"We can actually see how the domain walls make decisions in real time, providing direct evidence of their stochastic behavior," Arava explained.
This imaging revealed three key contributors to the randomness influencing domain wall motion: the topology of the injected domain walls, the geometry of the junctions and the strength of the applied magnetic field.
Topology refers to properties of the magnetic structure within the domain wall, which can influence its behavior and interactions. The researchers found that the direction of circulation of the magnetic vortex (clockwise or counterclockwise) in the domain wall can determine whether that domain wall goes left or right at the junction.
In nanomagnetic Galton boards, the junctions refer to the Y-shaped intersections where the domain walls encounter a decision-making point. This study showed that the size and shape of the junctions affect the complexity of the domain wall that the junction can support. Larger vertices can support a more complex domain wall, contributing to the randomness in path selection.
A magnetic field is used to drive the domain walls through the structure. The strength of this field was found to influence randomness by affecting the behavior and movement of the domain walls. Further, there is a critical field strength, known as the Walker Breakdown threshold.
Below this threshold, the domain walls move steadily through the structure and maintain their topological properties. But above this threshold, domain walls can undergo precessional motion, where the magnetic moments within the walls rotate around the direction of the applied field. This leads to more complex and less predictable behavior, increasing randomness.
Toward energy-efficient computing
By harnessing the natural randomness of domain wall motion in magnetic materials, researchers aim to create energy-efficient computing systems capable of complex decision-making tasks with minimal energy use. Unlike traditional computing, which often requires additional components to produce randomness, this approach leverages the intrinsic properties of the material.
"Our findings open up exciting possibilities for the future of computing," said Amanda Petford-Long, Materials Science division director and director of the Argonne Microelectronics Institute. "This work exemplifies the innovative research we are pursuing at Argonne to push the boundaries of microelectronics and develop technologies that can meet the growing demands for energy-efficient computing."
More information: H. Arava et al, Real Space Imaging of Field-Driven Decision-Making in Nanomagnetic Galton Boards, Âé¶¹ÒùÔºical Review Letters (2025).
Journal information: Âé¶¹ÒùÔºical Review Letters
Provided by Argonne National Laboratory