October 8, 2019 feature
High thermoelectric performance in low-cost SnS0.91Se0.09 crystals
![(A) A typical crystal cleaved along the (100) plane, and sample cut along b-axis. (B) A diagram shows how samples cut along b-axis for measurements. (C) Standard Laue diffraction image of SnS crystal along [100] direction. (D) Experimentally obtained Laue diffraction pattern of SnS crystal along [100] direction. The in-plane (b-c plane) directions of SnS crystal can be determined by using the standard diffraction image as a reference. Credit: Science Advances, doi: 10.1126/science.aax5123 High thermoelectric performance in low-cost SnS0.91Se0.09 crystals](https://scx1.b-cdn.net/csz/news/800a/2019/highthermoel.jpg)
Thermoelectric materials technology can convert between heat and electricity within a materials construct, but many existing materials contain rare or toxic elements. In a new study on Science, Wenke He and colleagues reported the temperature dependent interplay between three separate electronic bands in hole-doped (SnS) crystals. The materials behaviour allowed synergistic optimization between effective mass (m*) and carrier mobility (µ), which the research team boosted by introducing selenium (Se).
By alloying Se, they enhanced the power factor of the materials from approximately 30 to 53 microwatts per centimeter per square Kelvin (µWcm−1 K−2 at 300 K) and lowered the thermal conductivity. The research team obtained a maximum figure of merit ZT (ZTmax) approximating 1.6 at 873 K and an average ZT (ZTave; dimensionless figure of merit) approximating 1.25 between 300 K to 837 K within SnS0.91Se0.09 crystals. The researchers introduced a strategy for bond manipulation, which offered a different route to optimize thermoelectric performance. The high-performance SnS crystals used in the work represented an important step toward developing low-cost, earth abundant and environmentally favorable thermoelectrics.
Thermoelectric technology allows the invertible conversion between thermal energy and electricity to provide an environmentally friendly route for power generation. The process can occur by harvesting waste heat or by . Materials scientists and physicists have determined the conversion efficiency of thermoelectric technology using the dimensionless figure of merit (ZT) for a given thermoelectric material. The parameters that determine the conversion efficiency of thermoelectric technology are intertwined, making the manipulation of any single parameter to improve thermoelectric performance a challenge. Researchers had already devised several strategies to improve ZTs, by optimizing power factors via , or .
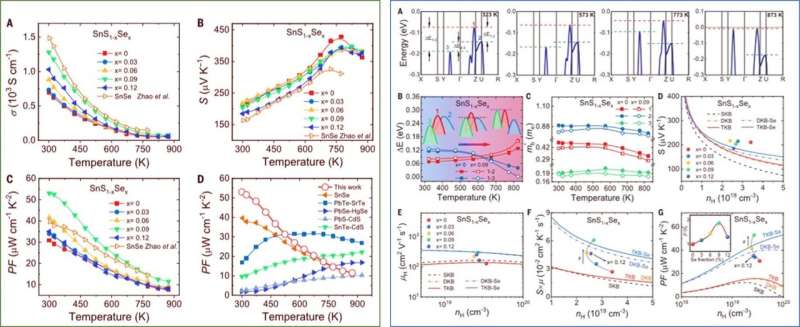
Scientists can decouple thermoelectric parameters by embedding and reduce thermal conductivity with . Materials scientists have also developed entirely new materials with intrinsically low thermal conductivity or with a large power factor, or with high-performance thermoelectrics sourced via reliable . High-performance thermoelectrics are typically widely studied across . The (Tin selenide) to the group is promising since thermoelectric materials do not contain these elements. Furthermore, SnSe has properties of alongside and three-dimensional (3-D) charge and .
The SnS compound is a structural analog of SnSe and predicted to be an attractive thermoelectric candidate as well. While the lower cost and earth abundance of S (sulfur) is appealing for frugal science and large-scale commercial applications, the low carrier mobility can cause poor electrical transport properties to impede high thermoelectric performance. In the present work, He et al. therefore explored the thermoelectric potentials of SnS crystals by , since the research team had also previously shown the ability to of SnS crystals. Since S was quite reactive with contact materials, it was important to develop a diffusion barrier in the future.
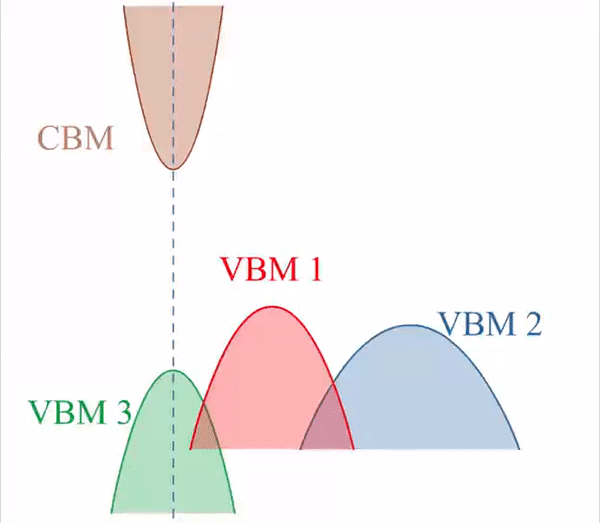
In the present work, the research team synthesized SnS1-xSex crystals using a temperature gradient method to investigate the role of Se in the compound. The team obtained temperature-dependent electronic band structures using density function theory calculations (DFT) based on atomic positions, which they derived using high-temperature (SR-XRD) data. Using the DFT calculations and measurements (ARPES), the team confirmed three separate electronic band interactions. They promoted the outstanding behavior interplay of the electronic bands by substituting S with Se to successfully optimize the effective mass (m*) and effective mobility (µ) within the material. They enhanced the power factor (PF) from 30 to 53 µWcm-1K-2 at 300 K. The team confirmed Se substitution using aberration-corrected (STEM) and (XAFS). Using (INS), He et al. showed that typical (acoustic waves) were softened by Se substitution and further coupled with acoustic branches for lower thermal conductivity.
The results further implied that the electrical conductivity improved due to enhanced carrier mobility after alloying 9 percent Se. The research team observed a combined increase in electrical conductivity and a large (thermoelectric sensitivity) to provide a PF (power factor) approximating 53 µWcm-1K-2 at 300 K for the SnS0.91Se0.09 crystals. The values were higher than those of other thermoelectric materials in the group IV to VI compounds. The research team schematically illustrated the dynamic evolution of the three valence bands and the energy offset between them as a function of temperature. Then by introducing Se, He et al. promoted the interplay of the three valence bands responsible to optimize the effective mass and mobility (m* and µ); where lowering m* resulted in improving µ.
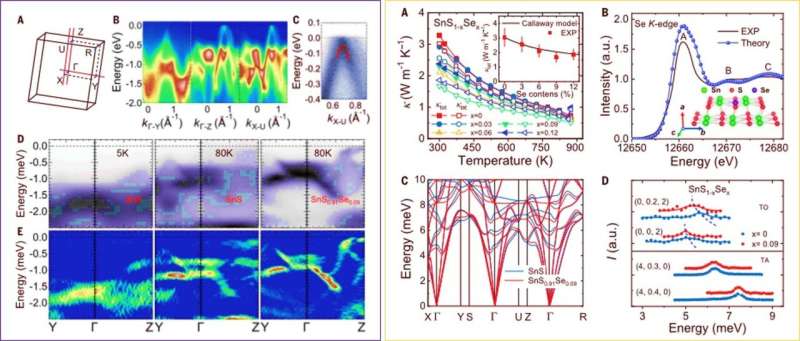
He et al. also used ARPES (angle-resolved photoemission spectroscopy measurements) to observe the electric band structure of SnS crystals. They plotted three valence bands along different directions and their relative energy levels in the (a theoretical zone). The scientists then conducted X-ray absorption fine structure spectroscopy (XAFS) on SnS1-xSex crystals to understand the Se substitution. Their work showed that for SnS0.91Se0.09 crystals, the (XANES) spectrum contained three main features. The research team reproduced all three major experimental features using a simulated spectrum and a Se substitution model. They observed the successful introduction of Se into the SnS lattice for all SnS1-xSex crystals.
![: Atomic-scale structures of high performance SnS0.91Se0.09 crystal. (A1, B1, C1) Atomically resolved STEM HAADF images along the [100], [010], and [001] zone axes, respectively, with enlarged images shown in the insets. (A2, B2, C2) The respective structural models. (A3, B3, C3) The respective electron diffraction patterns. (D) Atomically resolved STEM HAADF image along the [001] zone axis, with enlarged images showing the intensity difference between Se-substituted S and the SnS matrix. (E) Intensity profile from the dashed line of (C1) showing the higher intensity of Se-substituted S, compared with the SnS matrix. Credit: Science Advances, doi: 10.1126/science.aax5123 High thermoelectric performance in low-cost SnS0.91Se0.09 crystals](https://scx1.b-cdn.net/csz/news/800a/2019/3-highthermoel.jpg)
The team used (HAADF) to and view atomic-scale Se substitutions on S sites within SnS0.91Se0.09 crystals. They obtained structural modes and electron diffraction patterns for SnS and SnSe in . The abnormal brightness on the S sites indicated Se substitutions. They combined exceptionally high power factor (PF) and low thermal conductivity to generate a maximum ZT (ZTmax), for the SnS0.91Se0.09 crystals. He et al. showed good thermoelectric stability for the high-performance crystals, where the crystals showed excellent stability after for 432 hours. Such irradiation resistance is important for radioisotope thermoelectric generators for .
Compared with , SnS materials were far superior relative to toxicity and elemental abundance. The researchers expect to further optimize contact materials for SnS during elemental substitution to obtain higher experimental efficiency with low cost and high-performance in the future. In this way, Wenke He and colleagues used SnS0.91Se0.09 crystals to extensively demonstrate the great potential for competitive, large-scale applications in thermoelectrics materials technology.
More information: Wenke He et al. High thermoelectric performance in low-cost SnS0.91Se0.09 crystals, Science (2019).
Wenyu Zhao et al. Magnetoelectric interaction and transport behaviours in magnetic nanocomposite thermoelectric materials, Nature Nanotechnology (2016).
Li-Dong Zhao et al. Ultralow thermal conductivity and high thermoelectric figure of merit in SnSe crystals, Nature (2014).
Journal information: Science , Nature Nanotechnology , Nature
Provided by Science X Network
© 2019 Science X Network