February 27, 2023 feature
Tissue engineering: Developing bioinspired multi-functional tendon-mimetic hydrogels
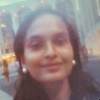
Thamarasee Jeewandara
contributing writer
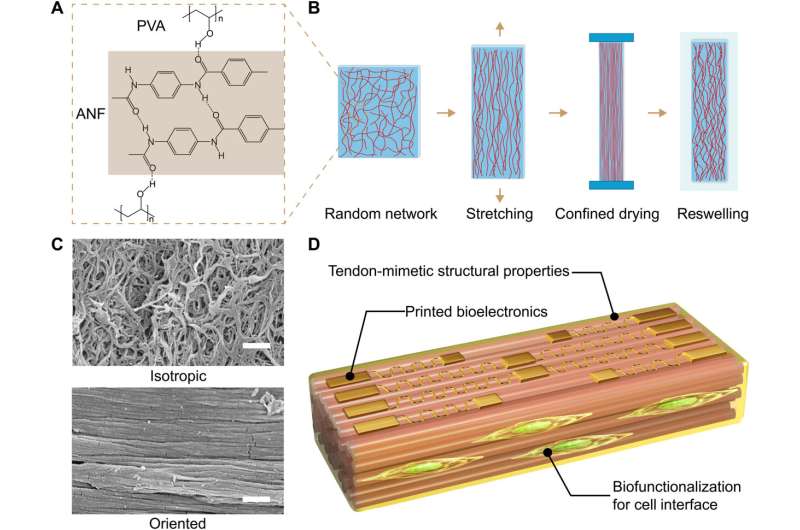
In a new report now published in Science Advances, Mingze Sun and a research team in physics, mechanical engineering, electrical and electronic engineering in Hong Kong China reported the development of multifunctional tendon-mimetic hydrogels by assembling .
The anisotropic composite hydrogels (ACH) contained stiff nanofibers and soft polyvinyl alcohol moieties to mimic biological interactions that typically occur between collagen fibers and in tendons. The team was bioinspired by natural tendons to develop hydrogels with a high elastic modulus, strength and fracture toughness.
The researchers biofunctionalized these material surfaces with bioactive molecules to present biophysical cues to impart behavioral similarities to those of cell attachment. Additionally, the soft bioelectronic components integrated on the hydrogels facilitated a variety of physiological benefits. Based on the outstanding functionality of the tendon-mimetics, the team envisioned broader applications of the materials in advanced tissue engineering to form implantable prosthetics for human-machine interactions.
Materials engineering a biomimetic tendon
Materials scientists work to develop advanced biological materials for medical devices and tissue engineering platforms to emulate natural biological tissue architectures via . However, the natural tissue architecture has a variety of characteristics that are difficult to synthetically replicate. The architecture of tendons relies on the load-bearing capacities of the musculoskeletal system to provide biophysical cues that translate into cellular behaviors via . In the past decade, researchers had devoted to engineer tendon-mimetic materials with high structural anisotropy.
Sun and colleagues developed an advanced materials platform for their work to construct hybrid anisotropic hydrogels with tendon-like behaviors and multifunctionality at the bio-interfaces. During the experiments, they established reconfigurable interactions between the stiff and flexible polymers to form a highly oriented framework that emulated a microstructural interplay between aligned collagen fibers and the soft proteoglycans. The biomimetic results of the anisotropic biophysical cues thereby regulated the cell behavior.
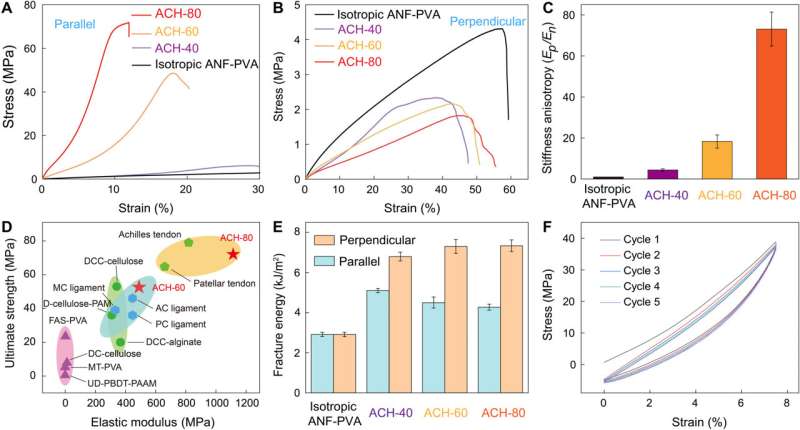
Creating an advanced polymer material in the lab
The research team developed anisotropic composite hydrogels by stretching and confining the material consisting of stiff and flexible polymer constituents. The resulting material demonstrated branched microstructures that mimicked .
The team conducted extensive hydrogen bonding between the two polymer constituents to create a three-dimensional network with high toughness where the fibrillar network did not undergo structural disintegration even under high levels of strain, leading to their . They then observed the characteristic fibrillar network of isotropic hydrogels that resembled hierarchical structures as seen in natural tendons—such efforts weren't as feasible with pre-existing synthetic hydrogels.
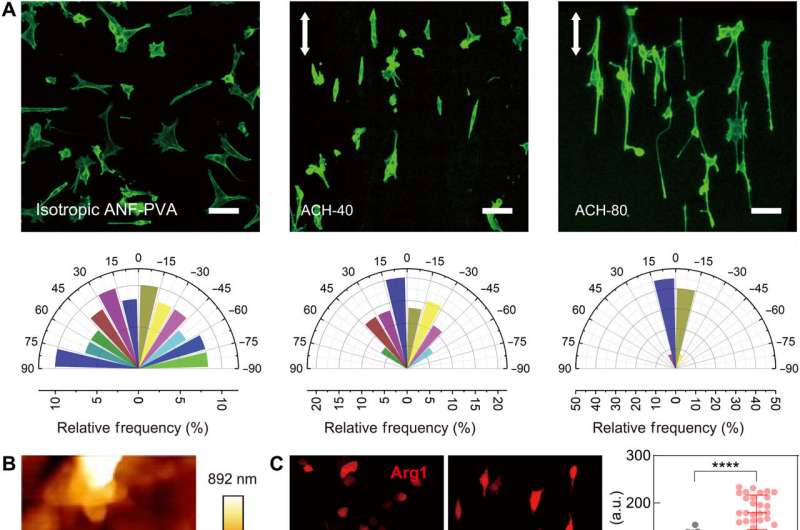
Biofunctionalization of the advanced polymers
The scientists next studied the structural characterization of the new polymer and its influence on cell behavior via interfacial interactions. They adopted chemical functionalization to present a cell adhesion motif such as the to bind with on the cell membrane. The researchers noted the successful biofunctionalization of the advanced materials by observing the adhesion of on the material surfaces, while samples without surface functionalization did not demonstrate similar cell attachment.
The (ROCK) molecules played a significant role by regulating the contractile machinery of cells during cell morphological responses to . The material constructs additionally regulated the between the pro-inflammatory M1 variants and the pro-healing M2 variants to establish bio-favorable implantable devices.
Advanced materials applications as bioelectronics
The research team ultimately demonstrated multimodal physiological sensing by integrating the advanced materials into soft bioelectronics. Among these iterations, they adopted a serpentine design to create wafer-based electronics with high stretchability to withstand the prewashing-drying process of the materials. They used finite element analysis to assess stress distribution across the device and enhanced the stretchability of the electronic component by modifying its for improved mechanical integrity.
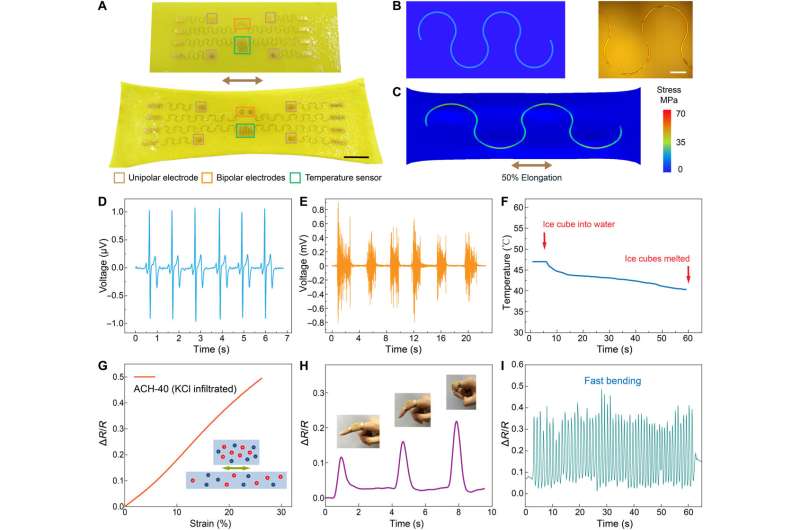
Outlook
In this way, Mingze Sun and colleagues engineered tendon-mimetic hydrogels with outstanding mechanics and functionality that predominantly originated from the assembly of nanofibers. They used biophysical cues presented by the materials constituents to regulate the cell dynamics, useful for advanced tissue engineering applications. The tendon-mimetic behavior of the advanced materials were useful as implantable tissue prosthetics.
The researchers examined the physical integration between the advanced materials and natural tissues in vivo and envision the possibilities of using multifunctional bioelectronics integrated on advanced materials. These include delivering critical capabilities such as physiological monitoring and integrating wireless modules for two-way communications between the external hardware and the electronically active prostheses.
Written for you by our author , edited by —this article is the result of careful human work. We rely on readers like you to keep independent science journalism alive. If this reporting matters to you, please consider a (especially monthly). You'll get an ad-free account as a thank-you.
More information: Mingze Sun et al, Multifunctional tendon-mimetic hydrogels, Science Advances (2023).
Jeong-Yun Sun et al, Highly stretchable and tough hydrogels, Nature (2012).
Journal information: Science Advances , Nature
© 2023 Science X Network