How 'spin currents' can be used to control magnetic states in advanced materials
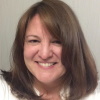
Lisa Lock
scientific editor
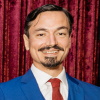
Robert Egan
associate editor

A new study reveals a fresh way to control and track the motion of skyrmions—tiny, tornado-like magnetic swirls that could power future electronics. Using electric currents in a special magnetic material called Fe₃Sn₂, the team got these skyrmions to "vibrate" in specific ways, unlocking clues about how invisible spin currents flow through complex materials.
The discovery not only confirms what theory had predicted but also points to a powerful new method for detecting spin currents—a discovery that could one day lead to more efficient memory and sensing devices in future electronics. The findings are in the journal Nature Communications.
Led by Assistant Prof. Amir Capua and Ph.D. Candidate Nirel Bernstein from the Institute of Applied Âé¶¹ÒùÔºics and Nano Center at Hebrew University in collaboration with Prof. Wenhong Wang and Dr. Hang Li from Tiangong University, the team explored how skyrmions behave in a special magnetic material called Fe₃Snâ‚‚ (iron tin).
This material is already known to be promising for use in advanced technologies because it keeps skyrmions stable even at extreme temperatures—a key requirement for practical devices.
What are skyrmions—and why do they matter?
Skyrmions are ultra-small, stable magnetic swirls that can exist in certain materials. Because they can be manipulated with very little energy, they are being studied as building blocks for future low-power memory and computing systems.
The team discovered that by sending electrical currents through Fe₃Sn₂, they could excite certain kinds of "resonances" in the skyrmions—essentially making them vibrate in very specific ways. These vibrations, or "modes," were detected using advanced optical techniques that observe changes in real time.
Interestingly, only two types of motion were triggered: a "breathing" mode (expanding and contracting like lungs) and a rotating motion. This confirmed earlier scientific predictions and suggests that Fe₃Sn₂ behaves differently than other magnetic materials.
A new type of spin current detected
The researchers also noticed something unexpected: The width of the resonance signal changed when they applied a steady current. Using computer simulations, they showed that this effect was caused by a "damping-like torque," which indicates the presence of spin-polarized currents. Furthermore, they realized that the resonances of the magnetic swirls were excited due to "spin-orbit torque" rather than the more familiar "spin-transfer torque."
"This gives us a deeper understanding of how spin currents interact with magnetic materials, especially in systems where the internal magnetic structure is frustrated or disordered," said Assistant Prof. Capua.
They also found signs that both real-space and momentum-space spin structures play a role in how electrons and spins move through the material, offering new clues about how to control electrical signals in future devices.
This research not only reveals new physics behind spin-torque effects but also opens up possibilities for using skyrmion resonances as highly sensitive detectors of spin currents—something that could benefit data storage, neuromorphic computing, and sensor technologies.
The study highlights how fundamental research in magnetism can lead to new tools for the electronics of tomorrow.
More information: Nirel Bernstein et al, Spin-torque skyrmion resonance in a frustrated magnet, Nature Communications (2025).
Journal information: Nature Communications
Provided by Hebrew University of Jerusalem