First-of-its-kind measurement may help physicists learn about gluons, which hold together nuclei in atoms
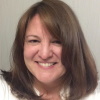
Lisa Lock
scientific editor
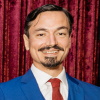
Robert Egan
associate editor

A team of physicists has embarked on a journey where few others have gone: into the glue that binds atomic nuclei. The resultant measurement, which was extracted from experimental data taken at the U.S. Department of Energy's Thomas Jefferson National Accelerator Facility, is the first of its kind and will help physicists image particles called gluons.
The paper revealing the results is and featured as an editor's suggestion in Âé¶¹ÒùÔºical Review Letters.
Gluons mediate the strong force that "glues" together quarks, another type of subatomic particle, to form the protons and neutrons situated at the center of atoms of ordinary matter. While previous measurements have allowed researchers to learn about the distribution of gluons in solitary protons or neutrons, they know less about how gluons behave inside protons or neutrons bound in nuclei.
"This result represents a big step forward in learning about where that gluon field is located in a proton," said Axel Schmidt, an assistant professor of physics at George Washington University and a principal investigator of this work. "We see evidence that it might be changing when a proton or neutron is inside a nucleus."
A charming guidepost particle
For more than four decades, the physics community has known that quarks, the building blocks of our visible universe, move slower when they make up a proton or neutron that's inside an atomic nucleus compared to a solitary proton or neutron. But physicists haven't been able to figure out why this phenomenon, known as the EMC effect, occurs.
To learn more about it, and the strong force in general, physicists need to probe gluons the way they probed quarks. However, measuring the distribution of gluons, which are neutral, is more challenging than measuring the distribution of electrically charged quarks.
"Studying these neutral particles that only interact by the strong force is much more difficult," said Lucas Ehinger, a graduate student at the Massachusetts Institute of Technology who worked on the analysis that led to this measurement. "We know a whole lot less about them and their dynamics in nuclei, including whether there's any kind of potential EMC effect with them."
This work takes a step toward changing this. And it does so by measuring a different particle altogether: J/ψ (or J/psi).
The experiment was carried out at Jefferson Lab's Continuous Electron Beam Accelerator Facility, a DOE user facility that supports the research of more than 1,650 nuclear physicists worldwide. CEBAF's electron beams can be used to also produce beams of high-energy photons for experiments that explore atomic nuclei.
Shooting a beam of photons at protons and neutrons can produce J/ψ particles, which each promptly decays into an electron and positron. Detecting this pair shows how many J/ψ were produced during an experiment. J/ψ is made of charm quarks. Because charm quarks, one of the six flavors of quarks, are not part of the proton or neutron, physicists know J/ψ is born from the interaction between the photon and gluon, which can produce particles containing any flavor of quark.
The production of J/ψ is a well-known tool for studying gluon distributions. Previous experiments at Jefferson Lab used a photon beam to measure J/ψ production of a solitary proton in the GlueX detector. To produce J/ψ, which is a heavy particle, the energy of the photon beam had to be very high—at least 8.2 GeV.
In this work, the experimental team also used a photon beam to produce J/ψ. However, they were able to do so using photons below the 8.2 GeV energy threshold and measure the results in the GlueX detector.
This was possible because they were using different nuclei as targets: deuterium, helium and carbon. Unlike a solitary proton or neutron target, the protons and neutrons inside these nuclei are moving around. Their kinetic energy combines with the energy of the incoming below-threshold photon, and, together, they offer enough energy to create J/ψ.
As a result, the team was the first to measure J/ψ photoproduction below the photon energy threshold required for a stationary proton. Because this measurement was taken off nuclei, it images the glue holding together protons and neutrons bound inside the nucleus.
"We are in this frontier of nuclear glue. Essentially, nothing is known, so everything you measure is informative," said Or Hen, professor of physics at MIT and a principal investigator of this work. "It's super exciting and super difficult at the same time."
Charting a map for future measurements
One reason this pathfinder measurement was difficult is because there weren't previous measurements to guide it. Embarking on the analysis, the team wasn't even sure it was possible. The experiment that collected this data wasn't originally supposed to measure subthreshold J/ψ production; it was more of a hopeful add-on.
Thankfully, the researchers had Jackson Pybus on their team. Pybus led the bulk of the analysis while he was a graduate student at MIT. He called upon his training during a summer abroad in Germany. There, he had worked with a theoretician to learn about light-front dynamics.
This approach generally applies to quantum field theories, which describe relativistic particle behavior (particles moving close to the speed of light). In this research, it is most useful in describing particle systems like nuclei. Applying tricks from this theoretical method allowed Pybus and the team to extract this measurement.
"This work is both unique in terms of what physics it teaches us, but also in terms of the techniques that a graduate student implemented to get at that physics," Hen said. "None of us, except for Jackson, would have been able to do this work. He deserves a lot of credit."
When the experimentalists compared their sub-threshold measurement to theoretical predictions, they saw that more J/ψ were produced than theory predicted. This disparity hints that the nuclear glue behaves differently than the glue in solitary protons or neutrons, but more data are needed to determine exactly how. Fortunately, these results will serve as a map to guide similar future measurements.
"Now that we know that we can do this measurement, we would really like to optimize a longer experiment to measure this in detail and quantitatively pin down some of these potentially exotic effects, where we really only have a first look right now," said Pybus, who is now a postdoctoral fellow at Los Alamos National Laboratory.
The data used in this work—made up of only dozens of J/ψ measurements– were collected during a relatively short six-week run in 2021. The team is proposing an experiment dedicated to studying the nuclear glue, again using CEBAF's photon beam with the GlueX apparatus.
"Imagine what we could do if we had 100 days of dedicated accelerator time to really study this reaction," Hen said. "We are now doing the very hard work of mapping out the frontier of the strong nuclear force with the hope that one day—maybe not in our lifetime—humanity's improved knowledge will enable better technology and more sustainable power sources."
These results could also steer gluon experiments at the forthcoming Electron-Ion Collider (EIC), which plans to further investigate gluons.
"We would like to learn about this problem and have a good handle of what's going on and where to look prior to this machine turning on," Schmidt said.
More information: J. R. Pybus et al, First Measurement of Near-Threshold and Subthreshold J/ψ Photoproduction off Nuclei, Âé¶¹ÒùÔºical Review Letters (2025). . On arXiv:
Journal information: Âé¶¹ÒùÔºical Review Letters , arXiv
Provided by Thomas Jefferson National Accelerator Facility